The global commercial drone market is anticipated to reach over $500 billion by 2028, with a CAGR of over 50%, and there is considerably increased demand for both commercial and personal use drones. Drone performance depends on many factors, which require design simulation to evaluate behavior and performance under actual conditions. SimScale is fundamentally a multiphysics simulation platform and supports a broad range of simulation physics applicable to drones and UAVs. A simple workflow in the SimScale platform can guide engineers through a drone/UAV simulation, showing the external aerodynamics using CFD and static structural simulation for loading and vibration analysis. New features such as automated mesh refinement zones can help users leverage cloud-native multiphysics simulation to analyze drones and UAVs. These features benefit engineers involved in developing and manufacturing drones/UAV propeller-based propulsion systems. This article describes the simulation setup and design insight for a quadcopter drone.
SimScale has multiple features useful to engineers designing and developing drones. Salient features include the ability to evaluate:
- Flow
- Aerodynamic loads under varying flight conditions
- Airframe-rotor-surrounding interference & impact on the stability
- Configurational effects on payload, endurance, and efficiency
- Aerodynamically optimized landing and take-off angles
- Structural
- Drone drop test
- Blade stress analysis
- Rotor shaft interference fit
- Vibration analysis
- Battery pack crush test
- Thermal Management
- Drone electronics cooling
- Early-stage battery pack design
- Battery pack cooling validation
Let us see how some of the above capabilities and features were applied to evaluate the performance of a quadcopter.
Simulating a Quadcopter
Starting with the airflow and aerodynamic performance, we can set up a CFD case to evaluate the quadcopter in hover mode. This is especially important to investigate as it thoroughly consumes the most power requirements. Drones in hover mode will also be in close proximity to the ground/roof or other objects and might be in confined spaces. Many applications will include drones entering or close to construction sites, mines, and homes for delivering packages and in urban areas where turbulence is more significant. Aerodynamic stability in hover mode is thus critical for the safety and overall performance of the drone and its surroundings.
We have taken a Parasolid CAD file of a standard quadcopter and made minor adjustments by removing the landing legs and camera mounts. This was done to simplify the geometry. Using the CAD editing feature in SimScale called CADmode, we can create the external fluid domain and further edit the geometry. In the first instance, we created rotating zones for the rotors with a rotational speed of 10,000 rpm. We have selected the Multi-purpose analysis type in SimScale, which lends itself to rotating machinery applications. The Multi-purpose analysis type includes a robust meshing strategy that produces an automated and robust hexahedral cell mesh, using the Cartesian meshing technique that significantly reduces mesh generation times by order of magnitude. It uses a finite volume-based solver optimized to handle a wide range of flow types. We have applied a globally coarse mesh with refinements around the rotating zones. Engineers and designers who evaluate the drone in hover mode will want to know its thrust performance, rotor-rotor, and rotor-airframe interactional effects. Selecting the analysis type automatically creates the simulation tree, a guided workflow for completing the simulation setup and post-processing the results. A user can now go through and set the material properties and boundary conditions and add rotational speeds to the rotating zones. The rotors are made of a polymer material (PVC); the fluid is air by default. Further time savings can be achieved by exploiting the symmetrical nature of the drone geometry and reducing the model size.
Using a transient simulation to capture time-dependent effects, we simulated for 200 minutes. Users can see velocity around the quadcopter and ascertain pressure to see forces in regions of interest, such as the rotors and joints.
We can run multiple scenarios of differing ground effect heights using further simulations. The ground effect in a multirotor is a change in the thrust generated by the rotors when flying close to the ground due to the interaction of the rotor airflow with the ground surface. It is critical for engineers to address the ground effect for safety and performance correctly. Steady-state simulations of the quadcopter take 20 minutes to run. Using the cloud’s computational power, several simulations can be set up and run in parallel, meaning the total time taken to complete dozens of simulation runs, for example, is still 20 minutes. When the quadcopter is closer to the ground, there is much more pressure on the drone’s underside, and the amount of thrust from the rotors is affected. It must have enough aerodynamic stability and structural integrity to still perform under varying forces, and this feedback is used intricately in the flight control system. Interestingly, we can see some wake air re-entering the rotors using velocity streamlines. This can cause safety problems in hover mode when close to the ground; for example, dust/dirt and other debris might enter the rotors/motors, causing damage.
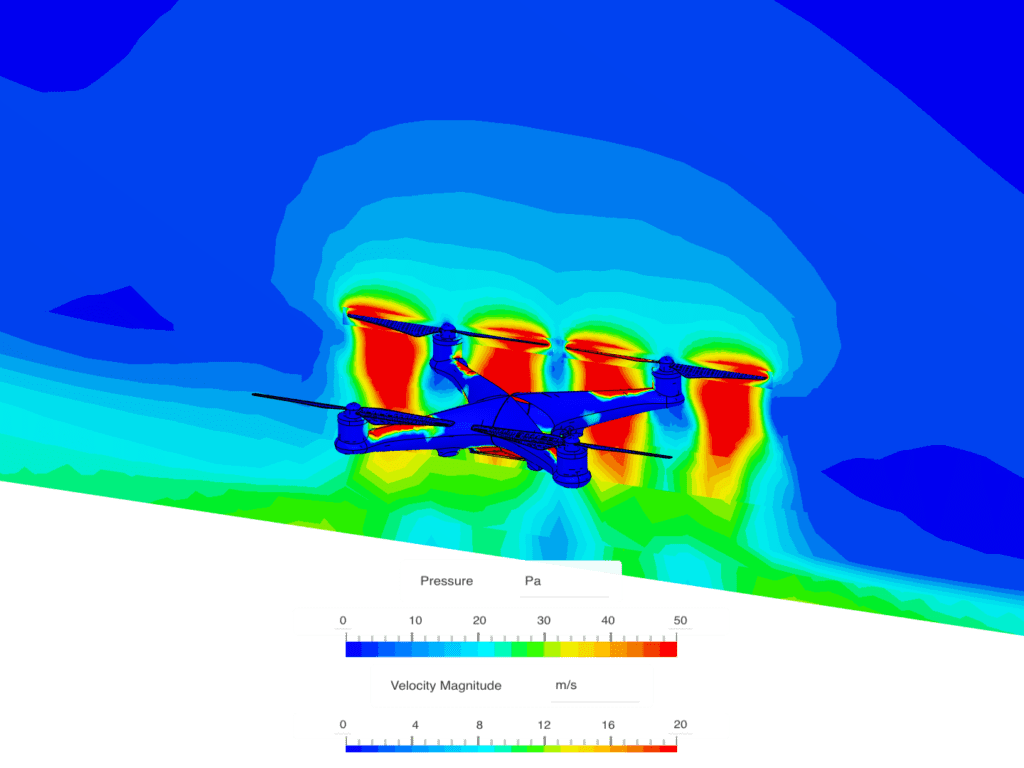
Analysis Type: | Multi-purpose CFD with body-fitted cartesian mesh |
Fluid: | Air at 200℃ |
Inlet boundary condition: | Pressure inlet |
Outlet boundary condition: | Pressure outlet/slip walls |
Flight regime: | Hover out-of-ground and in-ground effect (H/R) |
Insights required: | Hover performance, thrust augmentation due to ground effect, rotor-rotor and rotor-airframe interactional effects |
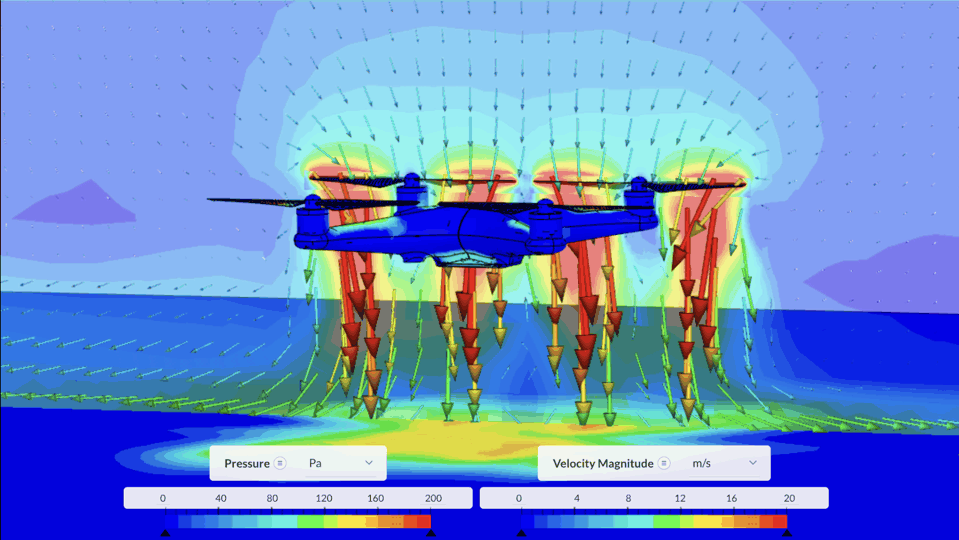
Drone power requirements change depending on rotor configuration. The number of rotors can impact thrust, drag, and overall performance. Engineers can investigate if a four or six-rotor aircraft might be better and the number of blades on each propeller. In this example, we can generate a force plot from the results and see a force 544N produced. For this study, we have compared the thrust outputs using SimScale to a published academic study showing experimental results and those from a competing CFD tool. The experimental results are shown in red, and the difference is attributed to geometry variations. SimScale results match closely with the competing CFD tool. The advantage of SimScale, however, is that the rate of change of operational thrust points on the curve can be simulated in parallel for each ground effect ratio, saving much time and cost.
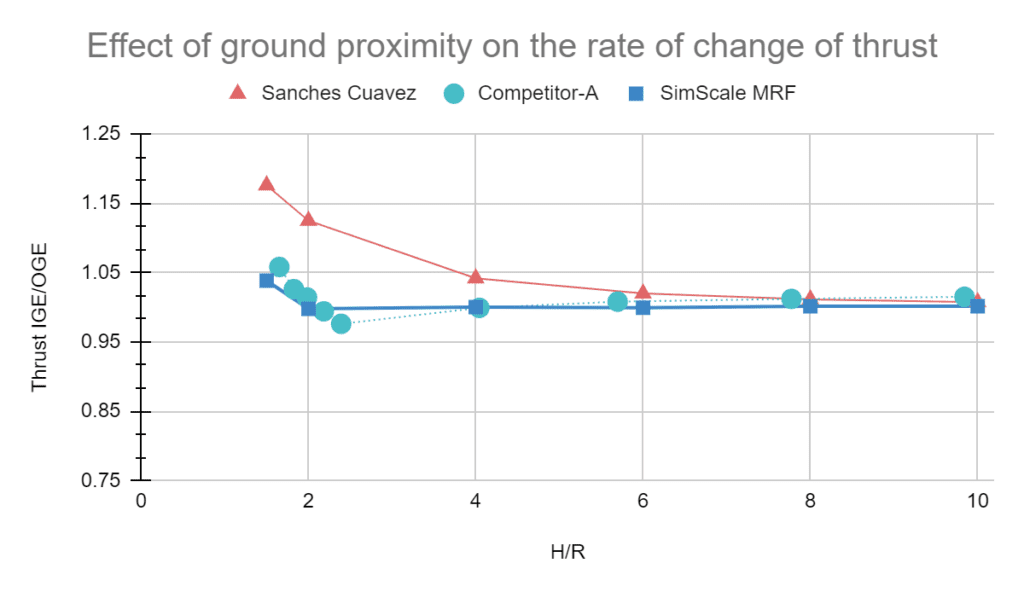
Flight Integrity
The structural forces on a drone can be considerable and change instantly. There are short-term structural effects like when in hover mode or landing; and longer-term material/joint fatigue and stress due to prolonged usage. Vibration analysis is critical to understanding fundamental structural integrity influencing flight stability and safety. Control systems must account for natural frequency and induced vibrational effects. With the right inputs, a flight control system can optimize the stability of a drone by avoiding specific rotor rotational speeds that might excite vibrations and cause adverse effects on the drone. Engineers must correctly apply vibration analysis to design stable drones over various rotational speeds. We have analyzed a propeller to evaluate its natural frequencies at 10,000 rpm and calculate the corresponding eigenfrequencies we wish to avoid. We can then run a harmonic analysis that accounts for these natural frequencies and the material’s damping properties to assess the propellers’ stress and displacement at those eigenfrequencies. For example, we can see high stresses at the rotor base that will cause fatigue over time.
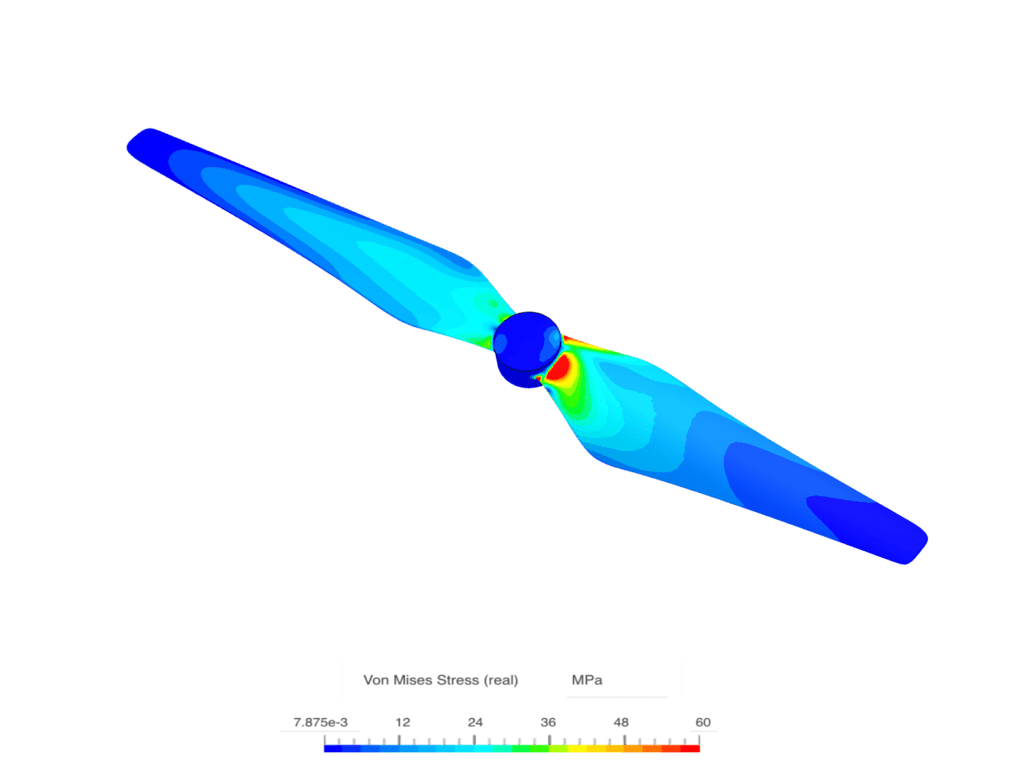
Engineers can also take the entire drone geometry and look at the global eigenfrequencies for the drone that might cause destabilizing movement. In this example, we calculated that 1630 and 6600 rpm would cause harmful effects due to adverse excitation of the drone. A Campbell diagram was used to find these values and represents a system’s response spectrum as a function of its oscillation regime. We have analytically calculated the eigenfrequencies as a function of shaft rotational speed. Essentially we plot the rotational speeds against the eigenfrequencies and read off where rotational speeds intersect the horizontal eigenmodes, which might cause excitation, and have found the intersection points occur at 1630 and 6600 rpm. For example, the rotor materials (PVC) and configuration can be changed to design around these problem points.
Analysis Type: | Frequency and harmonic |
Fluid: | Air |
Inlet boundary condition: | 10,000 rpm |
Outlet boundary condition: | Fixed to shaft |
Flight regime: | PVC, ABS |
Insights required: | Vibration analysis due to gyroscopic effect, natural oscillation modes |
Drone Flight Simulation
CFD has been helpful in simulating complex aerodynamics, evaluating drone performance & stability and visualizing airflow behavior. Structural analysis has allowed us to generate a Campbell diagram for vibration analysis, evaluate blade stress and durability and assess drone structural integrity. All these capabilities and many more are available to engineers through a web browser, and existing template projects make it easier to start.
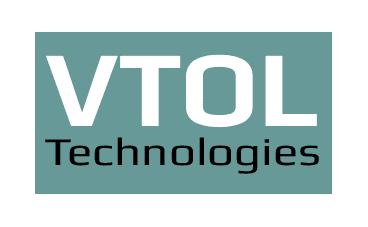
“We realized that some of the simulations of our drone design could be run much quicker and better with SimScale. Also, we used to not have a good process established, but now with SimScale, we have a proper design methodology and a validation tool for all the design modifications and improvements.”
— Marta Marimon, Aeronautical & Flight Mechanics Engineer at VTOL Technologies
This on-demand webinar helps teams involved with drone/UAV research and development learn how to test their products for desired aerodynamic performance and structural integrity with cloud-native CFD simulation:
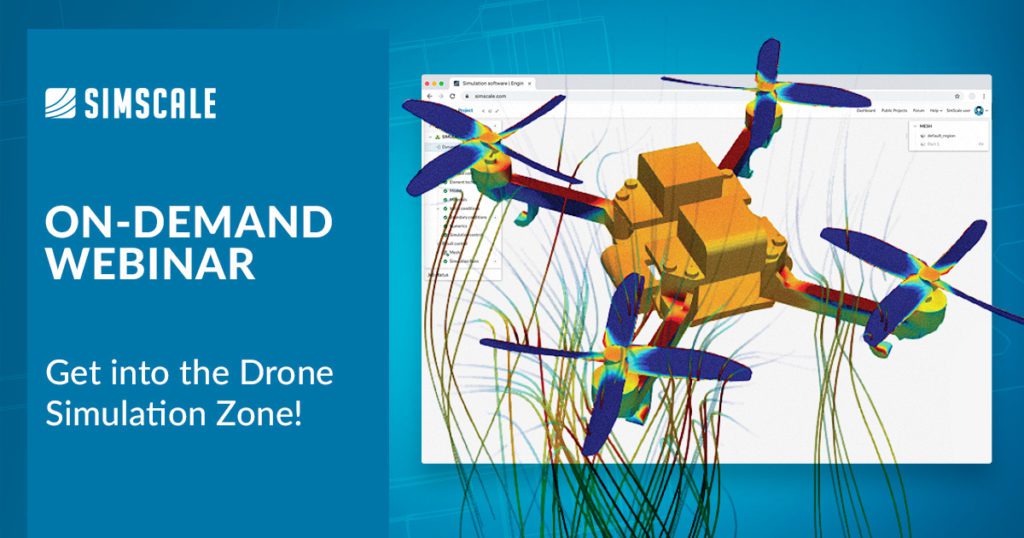